This bacterium could change the world: scientists have high hopes for plastic-eating microorganisms
In 2001, a group of Japanese scientists made a startling discovery at a garbage dump. In trenches filled with dirt and waste, they found a slimy film of bacteria that happily chewed on plastic bottles, toys and other small items. By breaking down the trash, the bacteria extracted energy from the carbon contained in the plastic and used it to grow, move, and divide into even more plastic-hungry bacteria. Even if it's not exactly how we usually understand it, the bacterium was feeding on the plastic. How this can change the world, the publication said The Guardian.
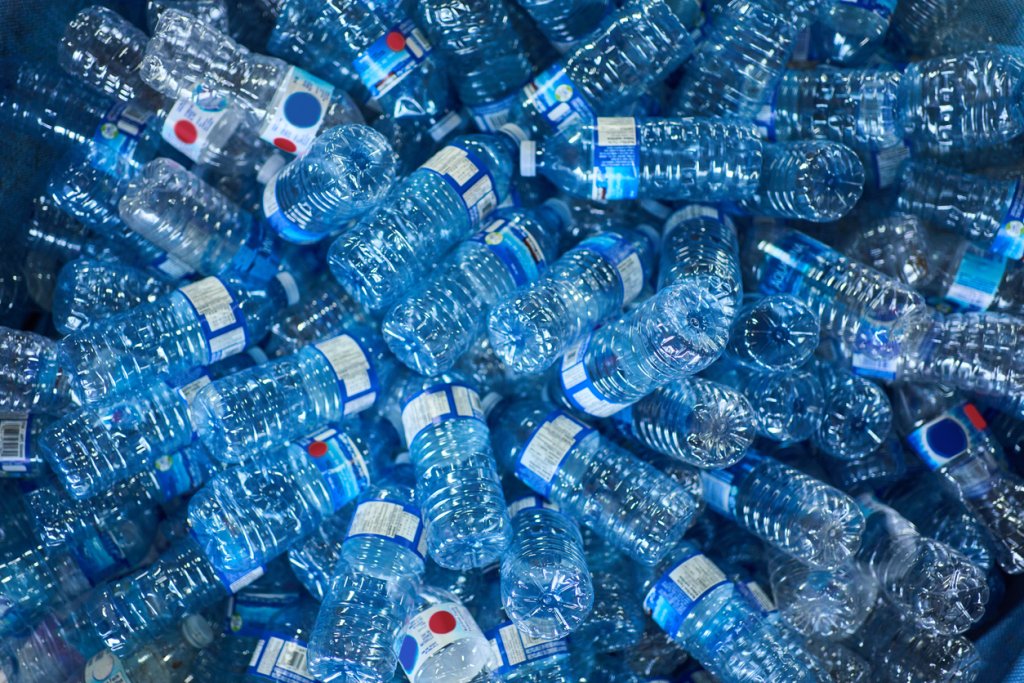
Photo: IStock
You just need to look
The scientists worked under the guidance of Kohei Oda, a professor at the Kyoto Institute of Technology. His team was looking for substances that could soften synthetic fabrics such as polyester, made from the same type of plastic used in most drink bottles. Oda is a microbiologist, and he believes that no matter what scientific problem a person faces, microbes have most likely already found a solution.
“I tell people, keep a close eye on this part of nature. She often has very good ideas,” Oda noted.
What he and his colleagues discovered at the landfill had never been seen before. Scientists hoped to identify a microorganism that had developed a simple way of attacking the surface of plastic. But this bacterium did much more—it seemed to completely break down plastic and convert it into essential nutrients. From our point of view, when we are well aware of the scale of plastic pollution, the potential of this discovery seems obvious. But in 2001 (three years before the term "microplastics" came into use), Oda says it "wasn't considered a topic of much interest." The preliminary work on bacteria collected by his group was never published.
In the years since the group's discovery, plastic pollution has become impossible to ignore. Over this roughly 20-year period, we have generated 2,5 billion tons of plastic waste and produce around 380 million tons more every year, with this amount projected to triple by 2060. The center of the Pacific Ocean is home to a plastic waste pile seven times the size of the UK, with plastic waste littering beaches and overflowing landfills around the world. On a miniature scale, micro- and nanoplastic particles have been found in vegetables and fruits, where they entered through plant roots. They are also found in almost all human organs - they can even be passed from mother to child through breast milk.
Current methods for breaking down and recycling plastics are woefully inadequate. The vast majority of plastic recycling involves a crushing and shredding step, which breaks down the fibers that make up the plastic. While glass or aluminum containers can be melted down and repurposed an unlimited number of times, smooth plastics such as water bottles break down with each recycling. A recycled plastic bottle becomes a colorful bag, which turns into fiber insulation, which becomes road filler and can never be recycled again. And that's the best case scenario. In reality, virtually none of the plastic—just 9%—ever reaches recycling plants.
On the subject: Drank coffee - eat a cup: a startup figured out how to save the world from plastic
The only permanent way we have found to dispose of plastic is by incineration, which accounts for about 70 million tons of plastic each year, but incineration contributes to the climate crisis because it releases the carbon contained in the plastic, as well as harmful chemicals with which it may be mixed, into the air .
For several years after his discovery, Oda and his student Kazumi Hiraga, now a professor, continued to correspond and conduct experiments. When they finally published their work in the prestigious journal Science in 2016, it went out into a world desperately seeking a solution to the plastic crisis and became a hit. Oda and his colleagues named the bacterium they found in a landfill Ideonella sakaiensis (after the city of Sakai where it was found) and described in the paper a special enzyme produced by the bacterium that allows it to break down polyethylene terephthalate (PET), the most common plastic used in clothing and packaging. The work received widespread press coverage and currently has over 1000 scientific citations, placing it in the top 0,1% of all papers.
But the real hope is that it's not just one type of bacteria that can feed on a particular type of plastic. Over the past half-century, microbiology (the study of small organisms, including bacteria and some fungi) has undergone a revolution that Joe Handelsman, former president of the American Society of Microbiology, called the most significant biological advance since the discovery of evolution. We now know that microorganisms represent a vast, hidden world intertwined with our own. We are just beginning to understand their diversity and often incredible capabilities. Many scientists have come to believe that for many of the seemingly intractable problems we work on, microbes may have already begun to find a solution. You just need to look.
"Two steps forward and one step back"
A discovery like Oda's is just a starting point. To have any hope of mitigating the effects of this global environmental disaster that we have created for ourselves, bacteria must work faster and better. When Oda and his team initially tested the bacterium in the laboratory, they placed it in a test tube with a piece of plastic film 2 cm long and weighing 20 grams. At room temperature, the bacterium broke down a tiny piece of plastic into its precursor liquid in about seven weeks. This was very impressive, but too slow to make a meaningful impact on the plastic waste problem nationwide.
Fortunately, over the past four decades, scientists have made significant advances in creating and manipulating enzymes. When it comes to chewing plastic, University of Portsmouth molecular biophysics professor Andy Pickford said, "The Ideonella enzyme is actually at a very early stage in its evolutionary development." The goal of scientists is to complete this path to the end.
When any living organism wants to break down a larger compound (be it a strand of DNA, a complex sugar, or a plastic), it turns to enzymes, tiny molecular machines inside cells specialized for the task. Enzymes help chemical reactions occur on a microscopic scale, sometimes by forcing reactive atoms closer together to bind them, or by twisting complex molecules at specific points to make them weaker and more likely to break apart.
If you want to improve the functioning of natural enzymes, there are approaches that work in almost all cases. For example, chemical reactions proceed better at higher temperatures, but most enzymes are most stable at the ambient temperature of the body in which they work—37 degrees, as is the case in humans. By rewriting the DNA that codes for an enzyme, scientists can change its structure and function to make it more stable, for example at much higher temperatures, which helps it work faster.
This power seems divine, but there are many limitations.
“It's often two steps forward and one step back,” says Elizabeth Bell, a researcher at the US National Renewable Energy Laboratory (NREL) in Colorado.
Evolution itself involves trade-offs, and although scientists understand how most enzymes work, it remains difficult to predict which changes will make them work better.
“Logic design tends to not work very well, so we have to use other approaches,” Bell admitted.
The work on PETase, an enzyme produced by Ideonella sakaiensis to break down polyethylene terephthalate, uses a brute force approach to speed up natural evolution. Bell takes the sections of the enzyme that directly work with plastic and, using genetic engineering, exposes them to all possible mutations. In nature, a mutation in an enzyme can occur only once in every few thousand divisions of a bacterium.
Bell guarantees that he will receive hundreds or thousands of potentially useful mutants for testing. She will then measure each one's ability to degrade plastic. All candidates who show even a slight improvement receive another round of mutations. NREL research team leader Gregg Beckham calls this "enzyme evolution." Last year, he published the results of his latest research: the PETase enzyme he designed is capable of degrading PET many times faster than the original enzyme.
But creating an enzyme suitable for such purposes is not simply a case of scientists tinkering until they got the perfect tool. Until Oda's work was published in 2016, no one knew about the existence of bacteria that could digest plastic. We now have one reliably documented case. Given that only a fraction of the microorganisms have been discovered so far, there may be a much better candidate.
This question has led to a boom in so-called bioprospecting. Like searching for gold in a river, bioprospectors travel the world in search of interesting and potentially useful microbes. In 2019, a team from Gwangju National University in South Korea took a construction drilling rig to a municipal landfill outside the city and drilled 15 meters beneath the garbage trenches to uncover decades of accumulated plastic trash. In it, Professor Soo-Jin Yeom and her students discovered a species of bacterium, Bacillus thuringiensis, which turned out to be able to survive using plastic bags as food. Yoma's team is currently studying what enzymes the bacterium uses and whether it can actually process polyethylene.
In the vast mangrove swamps off the coast of Vietnam and Thailand, Simon Cragg, a microbiologist at the University of Portsmouth, is searching for other potential polyethylene-eating microbes.
“The plastic-degrading enzymes we have observed so far are very similar to the natural enzymes that break down plant leaf coverings,” he said. “Mangroves have a similar waterproof coating in their roots, and swamps unfortunately have a shocking amount of plastic entangled in them.”
A microbiologist hopes that bacteria that can decompose mangrove roots will be able to switch to plastic.
Outdated science
For the nearly 200 years that we have been seriously studying them, microbes have been in a kind of scientific prison: they were mostly considered pathogens in need of extermination, or mere workhorses for a few basic industrial processes, such as the fermentation of wine or cheese.
“Until 40 or 50 years ago, microbiology was considered an outdated science,” said Handelsman, former president of the American Society of Microbiology.
In the XNUMXth century, as physicists made progress in splitting the atom and biologists came to classify many species of plants and animals, scientists studying very, very small areas of life lagged behind. However, there have been tantalizing signs of a hidden world beyond our reach.
Back in the 1930s, microbiologists puzzled over how there was a discrepancy between the world of microorganisms they encountered in nature and what they could study in the laboratory. Researchers have discovered that if you put a sample (a drop of seawater or a smear of mud) under a microscope, you can see hundreds of amazing and diverse organisms. But if you place the same sample in a gelatin nutrient suspension in a Petri dish, only a few individual species will survive and grow. When the researchers counted the number of colonies of microorganisms growing on the plate, it turned out that this was an insignificant handful compared to what the microbiologists had just seen in enlarged form. This was later called the "great plate count anomaly."
“With a microscope and then an electron microscope, you could see all these species. But they didn’t grow on plates, which is how we could characterize and study them,” explained William Summers, a physician and historian of science at Yale University.
Just like rare and exotic animals that cannot thrive in captivity, most microorganisms did not seem adapted to life in the laboratory. Therefore, scientists were limited to what could survive in their limited conditions. And yet there were microbiologists who tried to break out of this straitjacket and discover the true scale of the microbial kingdom.
The story of Alexander Fleming's discovery of penicillin in 1928 is well known: a fungal spore that swept through the corridors of St. Mary's Hospital and accidentally landed in Fleming's petri dish contained penicillin, which became one of the most powerful medical weapons of the XNUMXth century.
Less well known, but no less significant, is the story of chemist Zelman Waksman of Rutgers University, who coined the term “antibiotic” after noticing that some soil bacteria produced toxins that killed or inhibited other bacteria with which they competed for food.
Waksman worked tirelessly to create the conditions necessary to grow these wild bacteria in his laboratory, and his efforts resulted in not only the second commercially available antibiotic, streptomycin, in 1946, but also five subsequent antibiotics brought to market. As a result, searching for antibiotic-producing microbes in the soil turned out to be much more fruitful than waiting for them to get into the laboratory. Today, 90% of all antibiotics come from the group of bacteria through which Waksman made his first discovery.
Efforts like Waxman's are relatively rare. It was only with the discovery of simple chemical methods for reading DNA sequences, first introduced in the 1970s but widely and commercially available since the mid-1980s, that the situation began to change. Suddenly, microbes under a microscope could be cataloged and identified by their DNA, which hinted at how they might grow and function.
“More than that,” says Handelsman. “The genetic diversity we observed was enormous. It turned out that these life forms, although outwardly very similar to each other, are in fact very, very different. It opened the door to understanding how much more there is out there.”
About 25 years ago, scientists agreed that there were probably fewer than ten million species of microbes on the planet; in the last decade, some new studies have put the number as high as a trillion, with the vast majority still unknown. Scientists have discovered microbes in our bodies that affect everything from our ability to resist disease to our mood. In the depths of the sea, they found microbes living in boiling thermal springs. Microbes that have evolved to break down fossil fuels have been discovered in crude oil deposits. The more we search, the more unusual discoveries we will make.
Because of their adaptability, microbes are ideal companions to our turbulent times. Microbes evolve in ways and at speeds that would have shocked Darwin and his contemporaries. Partly because they divide quickly and can have populations of billions, and partly because they often have access to evolutionary tricks unknown to more complex life forms, such as the rapid exchange of DNA between individuals. They found a way to thrive in extreme conditions. And at this moment in history, man is creating increasingly extreme conditions around the world at an alarming rate. Where other animals and plants cannot find solutions quickly enough to adapt to their changing environment, microbes quickly adapt. They thrive in acidified water and have been shown to chew up some of the nasty chemicals we dump into nature. As Kohei Oda predicted, for many problems of our own making, they come up with their own solutions.
Holy grail
Finding new microbes and experimenting with them in the laboratory are the first steps, but scientists know that the final leap into what is commonly called the “real world” or “industry” can be elusive. In the case of plastic-eating microbes, this leap has already been made. Since 2021, the French company Carbios, using a bacterial enzyme, is processing about 250 kg of plastic waste every day, breaking it down into precursor molecules, which can then be used directly to produce new plastic. It's not exactly composting, but Carbios has achieved something like the holy grail of plastic recycling, bringing it closer to endlessly recyclable materials like glass or aluminum.
The plastic is first shredded, then put through a machine that resembles a huge press, which freezes it and forces it through a tiny hole under great pressure. The plastic comes to the surface in the form of granules (or, as they are also called, nurdels) the size of a corn grain. At the microscopic level, nurdel has a much lower density than what chemists call its original "crystalline" form. The fibers that make up plastic were originally packed into a tight lattice that made them smooth and strong; now, although the fibers are still intact, they are further apart and inactive, giving the enzymes a larger area to attack.
In nature, bacteria produce a limited amount of an enzyme that targets plastic, as well as a variety of other enzymes and waste products. To speed up this process, Carbios pays a biotech company to collect and concentrate huge amounts of pure plastic-digesting enzyme from bacteria. Carbios scientists then place the plastic nurdels in a solution of water and enzyme in a sealed steel tank several meters high. In a nearby laboratory where the process is being tested, the reaction can be observed in small containers. Inside, pieces of whitish plastic swirl around like flakes in a snow globe. Over time, the plastic breaks down, its components dissolve in the solution, and all that remains is a grayish liquid bubbling behind the glass. Instead of containing solid PET, the liquid now contains two liquid chemicals—ethylene glycol and terephthalic acid—that can be separated and converted into new plastic.
The technology developed by Carbios appears to be easily scalable. Two years ago, the company processed several kilograms of plastic in the laboratory, and now it processes about 250 kg per day. A much larger plant will open in 2025 near the Belgian border, capable of processing more than 130 tons per day.
The reason France has an operating plastic recycling plant using bacterial technology, while the US and China do not, is because the French state has made the problem of plastic waste a priority, with a target that by 2025 all plastic packaging used in France will be recycled. has been completely redesigned.
These plants are not a magic solution. The enzyme processing process is a series of biological and chemical reactions, and as production scales up, you discover that nature is a ruthless accountant. When you look at the various costs and carbon emissions involved, it turns out that cleaning plastic, heating it and freezing it requires significant energy costs. The chemical reaction itself causes the surrounding solution to become acidic, so as with an outdoor swimming pool, a chemical base must be constantly added to the solution to keep it close to neutral, resulting in several kilograms of sodium sulfate being produced as a by-product each time it is run reactions. Sodium sulfate has many uses, including in glassmaking and detergent production, but all steps from producing the chemical base to the subsequent use of sodium sulfate involve additional environmental costs and logistical challenges.
Emmanuel Ladent, CEO of Carbios, said the company's plastic recycling process currently reduces emissions by 51% compared to producing new plastic.
“Very good,” he concluded. “But I hope this is just the beginning.”
Carbios has not published the results of its analysis, but several other scientists familiar with the field said cutting emissions in half is a best-case scenario for this kind of recycling.
Carbios and the scientists behind it, University of Toulouse biologists Alain Marty and Vincent Tournier, have been working in this field for more than ten years. While many other scientists began doing similar research after the publication of Oda's discovery, Marty and Tournier began their work in the mid-2000s. They used a different enzyme called leaf compost cutinase (LCC), which has not evolved to work with plastic but could potentially do so, according to Marty and Tournier.
“It was a little weak and didn’t perform well in high temperatures, but it was a good start,” Marty explained. “After countless rounds of genetic engineering, the enzyme is clearly working.”
Gregg Beckham of the NREL research group says LCC is a "great enzyme." However, the researcher warns that it is not yet perfect. He prefers highly processed plastics and does not know how to work in the acidic environment that his own reactions create. Beckham hopes that since the enzyme produced by Ideonella Sakeinsis likely evolved specifically to fight plastic, it will be the best.
What's next
Can advanced microbes save us from the plastic crisis? Some scientists believe the technology will be limited. A recent critical review published in the journal Nature noted that many plastics will likely never be efficiently digested by enzymes because their chemical bonds require a relatively large amount of energy to break.
Andy Pickford, a professor at Portsmouth, is aware of these limitations but believes many potential targets still exist. “Nylon is a difficult but doable task,” he assured. “Polyurethanes too.”
You may be interested in: top New York news, stories of our immigrants and helpful tips about life in the Big Apple - read it all on ForumDaily New York
Scientists at Carbios agree and predict that they will develop a process for recycling nylon within a few years. If these predictions come true, about a quarter of all plastics will become truly recyclable; If it turns out that enzymes are suitable for all plastics that can theoretically be broken down, then just under half of all plastic waste could be recycled.
Even so, most scientists are aiming for a world in which enzymes can turn old plastic into new. It's depressingly limited. It makes economic sense, but it still involves producing plastic and consuming energy to do it. And while recycling may slow down the production of new plastic, it won't help us reclaim the unfathomable amount of plastic we've already released into the world, much of which remains too common, complex and dirty to reuse.
No microbes have yet been found that can truly transform a raw piece of plastic the way they transform organic matter: starting with a pile of carbon, say a human body, and leaving nothing but indigestible skeletal bits over the course of a year or so. When scientists find plastic-eating microbes on bottles in a landfill or on rafts of trash in the ocean, the best they can do is get a little nibble. Like a baby teething, they can't have much impact on anything that isn't softened and spoon-fed to them.
However, microbes have the power to destroy some of the planet's most harmful toxins, cleaning up entire landscapes in the process. This is best accomplished by chemicals that have been present on Earth for millions of years. When the Exxon Valdez dumped 1989 million liters of oil into the Gulf of Alaska in 41, coverage of the cleanup focused on images of environmentalists scrubbing oil from seals. However, much of the oil removal work has been done by bacteria that naturally feed on crude oil. About 50 kg of nitrogen fertilizers were spread along the coastline to promote bacterial growth. Similarly, when a former industrial site in Stratford was chosen to host the 000 Olympic Games, the committee tasked with cleaning it up transported more than 2012 truckloads of soil contaminated with oil and other chemicals to the sites where it was pumped into it over several weeks. nitrogen and oxygen, which caused rapid growth of bacteria that absorb toxins. The site was returned to Stratford and is now the site of the Olympic Park.
The question of whether the same can be done with plastics in the environment has received much less interest and funding.
“There is no market incentive to clean up our waste, be it CO2 or plastic,” said Victor di Lorenzo, a scientist at Spain's National Center for Biotechnology in Madrid and an advocate for the large-scale use of microbes to solve humanity's problems. — Recycling plastic pays off. But who will pay for these larger projects that will help the entire society? This can only be corrected with the help of public support.”
In addition to the market problem, there is also a legal one. In almost all countries, after the creation of genetic engineering of microorganisms, their release into nature without special permission, which is issued extremely rarely, is prohibited. The reasons for this are obvious. In the 1971 science fiction story Mutant 59: The Plastic Eater, a virus with the ability to instantly melt plastic spreads throughout the world, sending planes off course and collapsing buildings. It's unlikely that plastic-eating bacteria would become so effective, but something like this could lead to catastrophic consequences.
Di Lorenzo is convinced that the danger of such a development is minimal.
“When the first GMOs came out, people turned away from them. The scientists behaved arrogantly. It seemed like it was all about dominating nature and making profit. But we have a chance to change this. We can begin a new partnership between science and the natural world. If we present this to people honestly, they will be able to decide whether it is worth the risk,” he stressed.
The idea of a deeper partnership with microbes is very promising. The EU is funding several groups to develop microbes and enzymes to turn plastic into fully biodegradable materials, rather than just new plastic. Last year, a German team created Ideonella sakaiensis PETase in seaweed, noting that it could be used in the future to break down microplastics in the ocean.
Oda is convinced that we haven't even touched the tip of the iceberg yet. When he and his colleagues first discovered Ideonella in a landfill nearly 20 years ago, it wasn't working alone. “As soon as I saw the film of microorganisms on the plastic, I realized it was a lot of microbes working together,” Oda said.
His team realized that while Ideonella was breaking down plastic into industrially valuable raw materials, other microorganisms were processing them into simple nutrients that the microbial community could use. It was a symbiosis. In a sense, a partnership. After this, Oda wrote several papers in which he indicated that microbial communities could be developed into a system for removing micro- and nanoplastics from soil. But few people were interested in this.
In our conversations, Oda repeatedly lamented the lack of truly game-changing ideas coming from people who would like to commercialize the discoveries he and his colleagues made. There was incredible excitement about a plant that could turn old plastic into new, and a much smaller one, it seemed, about a plant that could turn plastic back into water and air.
Read also on ForumDaily:
Deceive scammers: a man earned $1782 by defrauding scammers
Batteries, paint, phones: how to get rid of 'hazardous' waste in the USA
From finding housing to naturalization: USCIS has released a Russian-language guide for immigrants
Costco members can get online doctor consultations for just $29
You can check your credit score weekly for free: how to do it
Subscribe to ForumDaily on Google NewsDo you want more important and interesting news about life in the USA and immigration to America? — support us donate! Also subscribe to our page Facebook. Select the “Priority in display” option and read us first. Also, don't forget to subscribe to our РєР ° РЅР ° Р »РІ Telegram and Instagram- there is a lot of interesting things there. And join thousands of readers ForumDaily New York — there you will find a lot of interesting and positive information about life in the metropolis.